3D printers enable us to build layer-by-layer small quantities of highly customized items. Items that fit to specified individual needs. Straight from a digital template, just designed with a computer aided design (CAD) software. With this technology you can quickly materialize customized tools, prototypes and any three-dimensional small parts. Really any?
The invention of 3D printing inspired bioengineers to think further and to look at it from a life science perspective. What about printing with biomaterial? With this thought the idea of 3D bioprinting was born. Gamechanging inventions start with some bold questions like these:
- What if we could print organic material?
- What if we could print organic cells?
- What if we could print organic tissue?
Membranes, skin or even organs?
Saving lives thanks to 3D bioprinting. Sounds fantastic doesn't it. But is it science fiction or is it real? Actually 3D bioprinting – 3D printing with organic (=living) material – is real, albeit still in its infancy. Microdrop is one of the early pioneers in microdosing and we have a lot of experience and expertise with it. Tinkering is in our engineering DNA. And 3D bioprinting is part of our microdrop life science business.
Are you are looking for technical solutions for your bioprinting project? Contact us and ask about the system family Gantry II from microdrop Technologies. We are happy to help.
Five current concrete advances
in 3D Bioprinting and its application for tissue engineering
How realistic is the idea of printing living material really?
There have been many recent advances in 3D bioprinting technology that have the potential to revolutionize tissue engineering.
To make it more tangible here are five concrete examples:
One major challenge in tissue engineering is creating vascularized tissues that can support the growth and function of cells. Researchers have developed 3D bioprinting techniques that can create vascularized tissues by using a combination of cell-laden hydrogels and sacrificial materials to create hollow channels that can be perfused with nutrients and oxygen.
Recent advances in 3D bioprinting have enabled the creation of complex tissues and organs, such as liver, heart, and lung tissue. Researchers have developed techniques to bioprint multiple cell types and create complex architectures that mimic the native tissue structure and function.
3D bioprinting allows for the creation of personalized implants that are tailored to the individual patient. This can be particularly useful for bone and cartilage implants, which can be bioprinted using a patient's own cells to reduce the risk of rejection.
Skin bioprinting has been used to create tissue-engineered skin for wound healing and cosmetic applications. Researchers have developed techniques to bioprint multiple layers of skin cells, including epidermis, dermis, and subcutaneous tissue, to create skin grafts that closely mimic natural skin.
3D bioprinting allows for the creation of complex tissue models that can be used for drug testing and disease modeling. Researchers have bioprinted tissue models of cancer, liver disease, and other conditions to study disease progression and test potential therapies. This is ethically much more attractive than research with living creatures (animals or human beeings).
Overall, the advances in 3D bioprinting technology have the potential to transform tissue engineering and regenerative medicine. 3D bioprinting technology enables the creation of complex tissues and organs, personalized implants, and tissue models for drug testing and disease modeling.
While 3D bioprinting was initially an idea from science fiction stories for literature, film and television, more and more effort is being put into realizing these visions. As mentioned above, initial successes have already been achieved. However, so far only basic forms of organs without functionality have been realized, so that further research is needed on the composition of bioinks and the 3D-Bioprinters themselves.
microdrop Technologies helps with highly precise, flexible and user-friendly technical systems and of course with professional advice and training.
Contact us. We are pleased to help.
3D bioprinting is an important research focus of regenerative medicine
3D bioprinting is very relevant for life science because it has the potential to revolutionize the field of regenerative medicine. Regenerative medicine aims to restore damaged or diseased tissues and organs using various techniques, including stem cell therapy, tissue engineering, and transplantation.
Traditional methods of tissue engineering and transplantation rely on obtaining tissues or organs from donors, which can be limited by availability, compatibility and ethical concerns. 3D bioprinting offers a potential solution to these challenges by enabling the creation of functional tissues and organs in the laboratory, using patient-specific cells and biomaterials.
Furthermore, 3D bioprinting enables precise control of the spatial distribution of cells and biomaterials, which is essential for creating complex, heterogeneous tissues and organs with the required structural and functional properties. This technology also enables the creation of in vitro models of human organs, which can be used for drug discovery, toxicity testing and disease modeling.
Overall, 3D bioprinting has the potential to significantly improve the outcomes of regenerative medicine and advance our understanding of human physiology and disease.
Let’s be real: Could 3D Bioprinting solve the problem of donor organs shortage or not?
3D bioprinting holds enormous potential. And research in the field of 3D bioprinting has already made significant progress. In this way, the basic shapes of organs can already be printed. However, 3D-Bioprinting is still in the early stages of development, and there are still significant challenges that need to be faced before fully functional organs can be printed for transplantation.
These challenges include ensuring that the printed tissue has the correct cell types, structure, and function to avoid immune rejection by the recipient. In the future, there will certainly be increased interest in the printing of functional blood vessels that enable the transport of nutrients and the preservation of cells and organs. Once this goal is achieved, research into the possibility of printing entire body parts using 3D bioprinting is within reach.
Additionally, 3D bioprinting may not completely eliminate the need for organ donation, as there may still be a need for certain types of organs that are not yet feasible to bioprint, such as the brain and the central nervous system. And to be honest: human biomaterial will never be completely replaceable. Human organ and tissue donation is still required at least as a source to produce individually suitable and compatible biomaterial in the laboratory.
In summary, 3D bioprinting has the potential to address the shortage of organs for transplantation, but further research and development is needed before it can become a viable solution.
One of the major current benefits for research: bioprinted experimental objects – instead of animal or human experiments
The possibility of studying life without having to resort to living beings is opened up by printing organs and other body parts. Diseases, medications and similar can be tested on individual, specially made test objects for this purpose without causing the death of living beings.
Fewer experiments on animals and humans are a positive side effect of the further development of 3D bioprinting. In this way, 3D bioprinting can be used to solve ethically relevant questions that focus on animal and human experimentation. The use of 3D bioprinting enables a reduction in animal and human testing, as the required test objects can be easily printed with the help of 3D bioprinting in the future.
Bioprinted Organoids and Organ-on-a-Chip are a recent preview of complete 3D Bioprinted functional organ
Due to the difficulties that still exist with 3D bioprinting of complete organs, organoids and so-called organ-on-a-chip (OoC) represent an important intermediate step in research.
Similar to the unit cell of crystal lattices, organoids are the smallest units that can still represent all functions of an organ. Organ-on-a-chip is a corresponding extension, in which small systems of organs and vessels are imaged on a substrate.
3D Bioprinted organoids and OoCs already offer the possibility to simulate reactions in the human body and of individualized medication
Organoids and OoC (Organ-on-a-Chip) promise a quick and easy way for research to map organ responses. For example, the reactions of organs to drugs can be simulated on a small scale.
Another possibility is individualized medication. This is invaluable and helpful, e.g. in the treatment of cancer patients. By using organoids and OoC, the tolerable and intolerable drugs for the patient can be determined and compiled accordingly by the medical staff.
3D Bioprinting (briefly) explained
In order to understand the process of 3D bioprinting and its challenges from a technical point of view, it is important to take a closer look at the bioprinting process and to highlight some special features. 3D bioprinting is an additive manufacturing process in which biological materials are built up in three-dimensional layers. The materials include living cells that are implanted in hydrogels.
In the bioprinting process, a 3D digital model of the structure to be printed is first created (using a CAD software). This 3D model is then loaded into the bioprinter. The bioprinter then precisely dispenses the bioink in a layer-by-layer fashion to create the desired structure.
The goal of 3D bioprinting is to create functional, living tissues and organs that can be transplanted into humans to replace damaged or diseased tissues.
This technology has the potential to revolutionize the field of regenerative medicine by enabling the production of customized, patient-specific tissues and organs that are less likely to be rejected by the body’s immune system. It could also reduce the need for organ donors and the risks associated with transplant surgery.
Hydrogels and Bioinks – Powerful magic ingredients
… with a highly sensitive mimosa-like character
Hydrogels are the scaffolding in bioprinting on which the structures are built. A hydrogel is a three-dimensional network of hydrophilic polymer chains capable of storing large amounts of water. In other words, hydrogels are natural and synthetic polymers that contain water while being insoluble in water. Hydrogels can be designed to have different physical properties, such as stiffness, elasticity, and porosity, by varying the type and concentration of the polymer used, as well as other factors like crosslinking agents.
Bioinks are special hydrogels used in 3D bioprinting. Bioink typically contains living cells, growth factors and other biomolecules required for cell viability and function. The bioink can contain different types of cells, including stem cells that can differentiate into various specialized cells such as muscle cells, bone cells, or skin cells, depending on the tissue of interest. Growth factors and other biomolecules support the cell growth and differentiation. The hydrogel matrix in the bioink provides structural support and enables precise deposition of the cells and other components.
Four important functions of hydrogels
To better understand the delicate matter and complex requirements of viable bioprints, let's look at four important functions of hydrogels:
1. Supportive structure:
Hydrogels can provide a supportive framework for cells grow and proliferate. They can be printed into complex structures that mimic the shape and function of native tissues, providing a scaffold for cells to adhere to and organize.
2. Cell encapsulation:
Hydrogels can also be used to encapsulate cells and protect them from the harsh environment of the printing process. This can help to maintain cell viability and promote cell differentiation, allowing the cells to form functional tissues.
3. Mimic native tissue properties:
Hydrogels can be formulated to mimic the mechanical and biochemical properties of native tissues. This can promote cell adhesion and differentiation and help to create a microenvironment that is conducive to tissue formation.
4. Biocompatibility:
The hydrogels used in bioprinting are biocompatible, meaning that they are non-toxic and do not cause an immune response. This makes them well-suited for use in tissue engineering applications, as they are less likely to be rejected by the body.
Overall, hydrogels are an important component of 3D bioprinting as they can provide a supportive structure for cells, promote cell differentiation and mimic the properties of natural tissues.
As we will explain later (see advantages of the piezo process), hydrogels, bioinks and cells are extremely sensitive. Their ability to survive and function is very dependent on the conditions in the immediate environment. Therefore, it is critical to monitor the conditions precisely.
What are the leading 3D Bioprinting technologies?
Various methods can be used to structure the hydrogel layers.
The most commonly used methods for 3D bioprinting are:
- Inkjet(-based) process
- Extrusion-based process
- Laser printing processes
Inkjet
3D Bioprinting:
Inkjet technology makes 3D bioprinting efficient (low cost, high speed) and gentle on the cells being printed (cell viability 85%). This technology uses inkjet nozzles to deposit small droplets of bioink onto a substrate to create the desired structure. With inkjet technology, the layers are built up by the targeted dispensing of bioink droplets. Advantages include high printing speed, versatility in printing different types of bioink and low cost. Probably the most significant benefit: inkjet 3D bioprinting is much gentler on the cells to be printed. Cell vitality is maintained, which is important for the survival and growth of the bioprint. Inkjet bioprinting works nearly without effect of heat, which is more likely to keep the cells to be dispensed alive. Of course, the cells are stressed by the shear forces whereas a cell viability of 85% cannot be achieved with any other method. The resolution here of 10-50 µm shows how accurate this technique works even if it is challenging to print complex structures.
Extrusion
3D Bioprinting:
3D bioprinting with extrusion-based technologies is extremely flexible in terms of material selection. The extrusion-based technology relies on the use of mechanical or pneumatic pressure. This kind of technology uses a syringe or nozzle to extrude bioink in a continuous stream to create the desired structure. Advantages include ability to print a wide range of biomaterials, and the ability to print large and complex structures. They can dispense almost all materials used for 3D bioprinting. And the resolution is relatively high (100 µm). But compared to the inkjet technologies, the resolution of the print result is significantly lower. So, the resolution is more on the side of the disadvantages. Other disadvantages are limited cell viability due to shear stress during printing, and potential clogging of the nozzle or syringe.
Laser-assisted
3D Bioprinting:
3D bioprinting with a laser printer impresses with its high resolution (10 – 100 µm). This technology uses a laser to vaporize the ink, depositing a layer of bioink on the substrate. The laser is used to selectively cross-link the bio-ink to create the desired structure. Advantages include very high resolution, ability to print complex structures, and the ability to create structures with high cell density. The main advantage of laser printing technology is the higher resolution. Disadvantages include limited printing speed and the need for specialized equipment.
JunJie Yu; Su A Park; Wan Doo Kim; Taeho Ha; Yuan-Zhu Xin; JunHee Lee; Donghyun Lee, Current Advances in 3D Bioprinting Technology and Its Applications for Tissue Engineering; Polymers 2020, Volume 12, Issue 12
Inkjet | Extrusion | Laser-Based | |
---|---|---|---|
Printer cost | Low(++) | Medium(+) | High(--) |
Print speed | Quick(++) | Slow(--) | Medium(+) |
Ink viscosity | 3.5-12mPa/s(-) | 6x107mPa/s(++) | 1-300mPa/s(+) |
Most used materials for bioprinting |
Collagen Alignate PCL |
Alginate GeIMA PEGDA |
Collagen Matrigel ECMs |
Formation time | Medium(+) | Low(++) | Long(--) |
Cell density | 85%(+) | 40-95%(++) | <85%(-) |
Resolution | 10-50 µm(++) | =100µm(+) | 10-100µm(++) |
Figure 2: modified according to 3D Bioprinting of Hydrogels for Cartilage Tissue Engineering by Huang, J.; Xiong, J.; Wang,
D.; Zhang, J.; Yang, L.; Sun, S.; Liang, Y.; Gels 2021, 7(3), 144; https://doi.org/10.3390/gels7030144“
Why inkjet-based 3D Bioprinting is the most promising bioprinting technology
Inkjet-based 3D bioprinting is the most promising technology that has several advantages over other 3D bioprinting methods.
Here are some of the special advantages of 3D bioprinting inkjet technology:
Inkjet bioprinters are capable of printing cells and biomaterials with high resolution and accuracy, enabling the creation of complex tissue structures with precise spatial control.
Inkjet bioprinters use a non-contact printing process, minimizing the risk of damage to delicate cells and tissues during the printing process and avoids cross contamination.
Inkjet bioprinting can be performed at high speeds and with high throughput, allowing for the creation of medium sized tissue constructs in a relatively short period of time.
Inkjet bioprinters can be used with a wide range of biomaterials, including hydrogels, extracellular matrix proteins, and synthetic polymers, giving researchers flexibility in tissue fabrication.
Inkjet bioprinting is a cost-effective method of 3D-Bioprinting, as it requires less materials than other bioprinting methods.
In combination, the advantages of inkjet technology for 3D bioprinting lead to a very high resolution of the print results with the efficient use of bioink at the same time. Inkjet technology thus offers further enormous potential for the future.
Groups of inkjet bioprinting technological approaches
Inkjet technology can be divided into different groups as shown in the following figure:
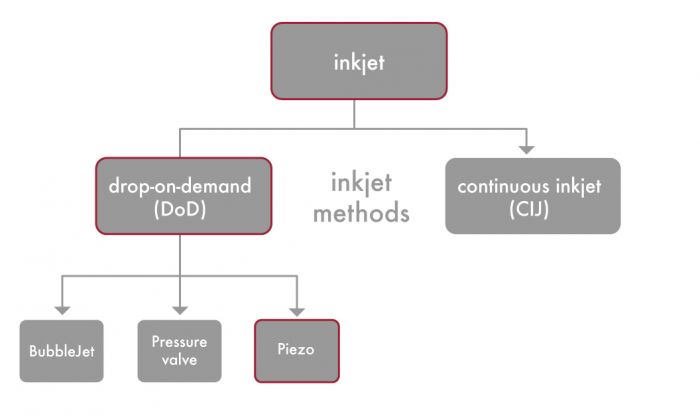
The two major groups into which the inkjet-based bioprinting systems are divided are drop-on-demand (DoD) and continuous inkjet (CIJ). The two groups differ fundamentally in the way the dosing of the bioink is performed. While CIJ devices use a continuous ink jet, the DoD bioprinters dispense individual drops with precise positioning onto the respective substrate. DoD systems are therefore characterized by a higher efficiency in using the required amount of the sometimes very expensive bioink.
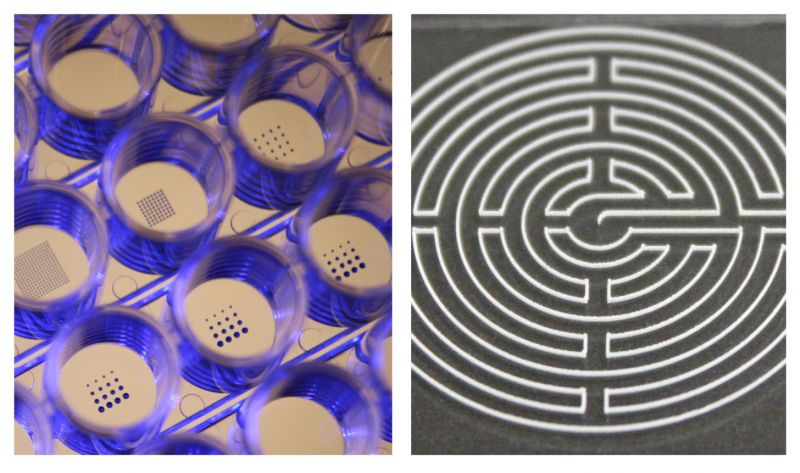
Challenges of DoD inkjet
One of the challenges of DoD inkjet printing is that the bioink must be carefully formulated to ensure that it can flow through the printhead nozzle without clogging, while maintaining its structural integrity and viability during the printing process. In addition, an accurate and reliable drop size is crucial to ensure that the printed structures have the desired properties.
Despite these challenges, DoD inkjet printing has shown promising results in the creation of various types of tissues, including skin, cartilage, and liver. Researchers continue to refine and improve DoD inkjet printing technology with the goal of creating functional and viable tissue constructs for transplantation and other biomedical applications.
To generate droplets,
the DoD Inkjet-based bioprinting offers 3 types of printheads to choose from. But a champion is just one of them.
In practice, within the drop-on-demand (DoD) inkjet-based printing systems three different types of printheads can be used to generate droplets. All these droplet generation methods can be applied for 3D bioprinting. Here they are::
- Bubblejet inkjet printing
- Pressure valve inkjet printing
- Piezo-inkjet printing
Bubblejet inkjet printing for use in 3D Bioprinting
With the BubbleJet, a heating element takes care of dosing the drops. Bubblejet printing is also known as thermal inkjet printing. It uses a printhead that contains a small heating element that heats the water or solvent in the bioink. This forms a small vapor bubble. The explosive pressure of this vapor bubble forces the bioink droplet out of the nozzle and positions it on the substrate. Bubblejet printing is a relatively fast and precise printing method, but it can be limited by the viscosity of the bioink.
Pressure valves in use to dispense a structure in 3D Bioprinting
In the pressure valve inkjet printing process, individual microvalves are attached to the nozzles in the printhead to control the flow of bioink onto the substrate. The microvalve is opened and closed by a computer-controlled actuator, which determines the pattern of bioink to be deposited. Printing with pressure valves is a highly precise and versatile printing method that can handle a wide range of bioink viscosities and create structures with high resolution.
Piezo-inkjet printing – The champion among inkjet solutions for 3D Bioprinting
Piezo-inkjet printing uses a piezoelectric crystal in the printhead to generate pressure waves that eject droplets of bioink from the nozzle. The piezoelectric crystal is activated by an electrical signal from the computer that determines the pattern of droplets to be applied. Applying an electrical voltage causes the element to deform, setting the ink in motion. By adjusting various parameters, the droplet and so its size can be set very precisely and the droplet can be dispensed accurately onto the substrate. Piezo-inkjet printing is a highly precise and versatile printing method, as it can handle a wide range of bioink viscosities and create droplets of varying sizes.
Piezo-inkjet printing is widely considered to be the best 3D bioprinting method for producing precise droplet dispensing and can therefore be declared the champion among the inkjet methods.
microdrop's piezo inkjet printing technology
is the champion for 3D bioprinting applications.
The nutrient supply for entire organs is highly complex. 3D Bioprinting requires extremely precise, coordinated interaction of hydrogels and cells.
Currently, the particular challenge with 3D bioprinting is the printing of a functional organ. To overcome this challenge, a framework for the shape and internal structure of the organ must first be available, analogous to the process described above. In a further step, the different cell types must be implanted in the right places. Finally, it is essential for a functioning organ to have a blood supply and thus a supply of nutrients. To achieve this, it must be possible to print blood vessels.
Although 3D bioprinting has made significant advances in recent years, there is still a long way to go before fully functional 3D printed organs can be produced for transplantation. Current research already demonstrates the feasibility of individual structures for nutrient transport. However, further efforts are needed in this area. An intermediate step is the printing of organoids.
Living cells are very sensitive. Therefore, precise controllability of the environmental parameters is required for 3D Bioprinting.
Another major challenge is the lifespan of the cells in the 3D bioprinting process. Cells are highly sensitive to environmental parameters, including temperature, pH, oxygen levels, nutrient availability, and mechanical forces. Even slight variations in these parameters can have significant impacts on cell behavior, including proliferation, differentiation, and apoptosis.
For example, temperature variations can affect the rate of enzymatic reactions and alter the stability of proteins and membranes, which in turn can affect cell viability and function. Changes in pH can also affect enzymatic reactions and alter the charge and conformation of proteins and other molecules, which can affect their activity and interactions.
Oxygen levels are also critical for cell survival and function, as many cellular processes require oxygen. Hypoxia, or low oxygen levels, can lead to cell death or dysfunction, while hyperoxia, or high oxygen levels, can also be toxic to cells.
Nutrient availability is also important for cell function, as cells require a steady supply of nutrients to carry out metabolic processes and maintain energy levels. Changes in nutrient availability can affect cell proliferation, differentiation, and function.
Finally, mechanical forces can also impact cell behavior, as cells can sense and respond to physical cues in their environment. For example, changes in the stiffness or elasticity of the extracellular matrix can alter cell adhesion, migration, and differentiation.
As cells are highly sensitive to their environment, researchers need carefully control and optimize the environmental parameters in order to create functional tissues and organs using tissue engineering and 3D bioprinting techniques.
Researchers need systems that can be used to monitor and control the following environmental parameters:
- temperature
- humidity
- pressure
Fluctuations in these parameters during 3D bioprinting must be avoided to keep the cells alive and to enable and enhance the printing of functional organs. With the Autodrop Gantry II systems from microdrop Technologies we offer the technology you need to monitor and control all these parameters. Contact us. We are happy to help.
The Autodrop Gantry II system is the perfect solution in terms of controllability of environmental parameters and flexibility for industry and research in the field of 3D Bioprinting
microdrop Technologies' Autodrop Gantry II system offers various add-on modules to control the environmental parameters that support 3D bioprinting, thus enabling the printing of living cells. As already mentioned, when dealing with the cells in 3D bioprinting, the humidity and the temperature on the substrate play a decisive role.
In the Autodrop Gantry II system, the humidity is controlled by the module AD-I-3010 “External ultrasonic humidifier”. With this, the humidity can be controlled and regulated in the system up to 80% RH.
The temperature on the substrate can be adjusted to the respective needs of the cells and organisms using an integrated heating or cooling circuit. We offer different sizes of vacuum clamping tables. Temperatures are possible from 10°C up to 100°C.
The team of experts from microdrop Technologies has many years of experience and know-how in dealing with scientific and engineering issues and thus enables creative individual solutions that are perfectly tailored to the needs of the user.
More details about customized configuration of the Autodrop Gantry II application >>>
The Autodrop Gantry II system is a flexible technical solution for applications in life science research and industry
microdrop Technologies provides the necessary flexibility on the development path with its Autodrop Gantry II configurations to meet a wide range of challenges. The Autodrop Gantry II system offers a variety of usable substrates. For example, free surfaces such as glass slides can be used and printed, but also hollow bodies such as microtiter plates. This flexibility in the choice of substrate offers enormous potential for use in 3D bioprinting of organoids and organs-on-a-chip.
The sterile maintenance of the dispensing systems and the mentioned use of different module configurations for environmental control should also be mentioned here. microdrop Technologies presents itself as a reliable partner for industry and research institutions.
Contact us. We are happy to help.
Beacon of hope: Can 3D Bioprinting soon mitigate the shortage of donor organs?
According to the Global Observatory on Donation and Transplantation (GODT) data published in 2020, there is a significant gap between the number of organs needed for transplantation and the number of available organs. The organs that are most urgently needed for transplantation vary by region and country, but in general, the most commonly transplanted organs include kidneys, livers, hearts, lungs, and pancreases.
Long waiting lists for organ donation …
It is difficult to give reliable data on exactly how many people are on organ donation waiting lists around the world. Data that can be found on the web varies greatly. The reasons for that are complex. The data is recorded decentrally and according to inconsistent criteria. In addition, the data is available under very different conditions, some completely open, some restrictive. They are also maintained and managed by various organizations and associations.
However, two things are undisputed:
- The need and the willingness to donate organs vary greatly from country to country.
- The demand for biological donor material (organs, cells, tissues etc.) is many times higher than the available biomaterial.
Organic resources are only available to a limited extent. The more specific the organic resource required, the more difficult it is to obtain. The possibility of creating living organisms with a 3D-Bioprinting process could fill countless resource bottlenecks. Especially in the regenerative medical field. Hardship, suffering and death can thus be significantly reduced.
The demand for organ transplantation has increased over the years due to the rising incidence of organ failure, chronic diseases, and aging populations. Despite the high demand for organs, the number of donors remains low. According to the WHO, less than 1% of deaths in hospitals occur in circumstances where organ donation is possible. This shortage of donors leads to long waiting times for patients in need of organ transplantation. By the way, corneas, heart valves and blood vessels are tissues that can also be transmitted from one person to another.
When a vital organ fails, often only a suitable donor organ can save that person’s life. But there are no organs in the supermarket. Organs are in short supply. To make matters worse, not every organ is compatible. The requirements are complex. Depending on the organ involved, living donation is not possible. Only a few organs are eligible for living donation. In Germany, these are primarily the kidneys and parts of the liver. However, parts of the lungs, small intestine and pancreas are also legally permitted as living donations, but are less common.
The unwelcome truth applies to all other organs: A suitable donor must die for the suitable donor organ to become available. In this context, the declining willingness to donate organs is problematic. So it’s no wonder that the issue of organ donation is under a lot of pressure. It is urgent, but also explosive and highly polarizing, in short: highly emotional. In any case, organ donation is a major challenge for everyone involved.
On the (german) website organspende-info.de you will find further education and information on the subject of organ donation. You can also order an organ donor card there for free.
Further Sources / other sources / link recommendations / other links of interest:
For global statistics and detailed information about organ donation and transplantation visit for example the Website of the Global Observatory on Donation and Transplantation (GODT).
The GODT is an initiative based on a joint of the WHO (World Health Organization) and the Spanish Transplant Organization ONT (Organización Nacional de Transplantes).
Another global Data-Collection we recommend is the International Registry in Organ Donation And Transplantation (IRODaT.
It’s an open Data Resource. Its statistics come from official reporters. Most of them are members of National Transplant Organizations and Ministries of health. There you get insights about organ donations and organ transplantations, filterable by country.
Contact us
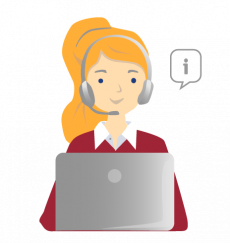
Whether you're looking for solutions,
facing challenges or just want to let us know what your next project will be.
We are there for you.